Nate Lewis is the George L Argyros Professor of Chemistry and Scientific Director of the Joint Center for Artificial Photosynthesis (JCAP) at Caltech. JCAP is one of several federally funded Energy Innovation Hubs, with a specific focus on deriving fuel from sunlight. In a recent talk given at the LAEDC Mid Year Economic Forecast, republished here in TPR, Professor Lewis puts the earth’s current greenhouse gas levels in historic perspective, noting that no energy source has the power to both clean our atmosphere and meet civilization’s growing energy demands except the sun. Yet harnessing the sun’s energy with current solar panel technology presents problems of space and storage. Lewis outlines how artificial photosynthesis might solve these problems, as well as the steps Cal Tech is taking to make this hypothetical technology a game-changing reality.
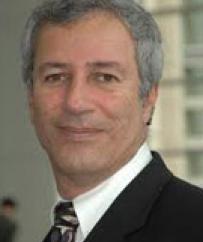
Nathan Lewis
We were so proud when we got a shout-out from President Obama during the State of the Union address when he said, “At Caltech, they’re taking water, sunlight, and carbon dioxide and making fuel for our cars.” That’s what we’re trying to do. -Nate Lewis
Energy is so big that it needs its own units to describe it. We hear a lot about barrels of oil, cubic feet of natural gas, and kilowatt-hours of electricity, but I’m going to bring it down to the unit that people can perhaps remember, and that is watts.
So how big is energy? Well, a laptop is a few watts. If you have 1000 laptops, you get a toaster; that’s a kilowatt.
A trillion watts is what the world’s electricity meter reads on average, all the time. It turns out that when you add in all the overall gas, oil, and coal we use, it’s a factor of ten more than that. So you can’t solve the energy problem unless you are talking on the order of the problem of energy, and right now the world’s energy meter reads at nearly 15 trillion watts.
All projections show that energy demand will double within our lifetimes due to population and economic growth. It would have tripled, except that we have efficiency built in, fortunately, to make better use of natural resources. If we save yet again as much energy as all the energy the world uses today, then energy demand will just be flat at 15 trillion watts. That’s what it takes to make a demand scenario in energy.
The Stone Age didn’t end because we ran out of stones. The fossil fuel era is not going to end any time soon because we run out of oil and gas. There are plenty of oil, gas, and coal molecules in the ground.
The gorilla in the closet is the uncertainty associated with getting 15 to 30 trillion watts of energy from predominately fossil energy. I am not personally for or against any individual energy technology. I am not personally for or against any outcome and don’t make policy. But I can tell you what we know and what we don’t know about the implications of continuing [with our current energy portfolio].
We know that the carbon dioxide in the earth’s atmosphere has been in a narrow band between 200 ppm and 300 ppm for 670,000 straight years. We know this by looking at ice core data and measuring the bubbles trapped in the ice year by year. We also know, from the earth’s rock records, that the CO2 levels now are higher than those in the past million years. We also know the earth’s temperature has changed with time and that the changes in CO2 levels correspond with when the world has gone into and out of ice ages, not once, but seven times. But correlation does not necessarily mean that the cause of temperature changes that have swung our planet into and out of ice ages is due to a 100-ppm change in carbon dioxide.
We know, by the laws of chemistry, that when we burn oil, coal, and gas, carbon dioxide is emitted. To give you a feeling for how much is emitted, imagine you drove your car a mile on the freeway and every mile every car stopped, opened the window, and emptied a pound of trash. That’s exactly what we do, because we emit, on average, one pound of carbon dioxide for every mile a vehicle drives on the freeway.
We know that that amount of consumption has lead to the increase in carbon dioxide in our atmosphere to now about 400 ppm, and we know that if we continue on the business-as-usual trajectory, we will be above 550 or 600 ppm in our lifetimes.
No one can tell you precisely what this will do. There are at least six major climate models, and they all differ from each other, which means we know that at least five of them must be wrong. We also know that we don’t know what exactly the climate was like 100 years ago—there’s no way to calibrate the models to know whether or not they are actually valid. We furthermore do this by averaging all of the models, but the earth isn’t on an average path—it’s on one path, and nobody knows what path that is.
This is not about our ability to definitively say what the impacts of taking our planet to a place that no human has ever lived in will be. It is absolutely about the fact that we know we have never been there before and we know that, seven for seven, excursions of a smaller extent have corresponded with our planet going into and out of ice ages. We also do know the lifetime of carbon dioxide in our atmosphere. If we stopped cold turkey and didn’t burn any oil, coal, or gas again starting in 2040, the CO2 would last, at that level, for the better part of 10,000 years. That’s how long it takes since there are no natural destruction mechanisms for carbon dioxide (it has to naturally dissolve in the ocean and go away into the rock). So we do know that one way or another, on this path, we are going to change our climate for a timescale comparable to modern human history. We don’t know whether that will be good, bad, or in between. We do know that we would have to bet against 670,000 years of data as a leading indicator and hope that we get lucky this time.
Now we do know that the earth is warming. We know that glaciers everywhere are melting. If you’re like me and go to Glacier National Park, you go to an area called Many Glacier, which had 230 glaciers in 1910—now it soon will be called Not So Many Glaciers. They won’t be there for long; they will all be gone within two decades or less.
We know that 20 percent of coral is already bleached because, in keeping with the laws of chemistry, when you add carbon dioxide to water you make it acidic. That’s how we make soda.
What does this all mean? Remember I told you that we generate 15 trillion watts of energy right now? If you wanted to stop not just the growth rate of CO2 emissions, but actually stop emissions so the earth could recover—and I’m not saying that we will do this, or that it will be cheap, or that the countries of the world will enact policies to actually do it—I’m just telling you the scientific arithmetic that would be involved if we decided that that is not where we want to go.
If you don’t want to go there, then you have to make as much clean energy as all the energy we use on the planet combined today—and that will just hold the energy demand constant. If you want to actually limit CO2, you have to make that demand equal to supply. That means that if you want to take fossil energy and not just burn it, but take the CO2 that’s emitted from the stacks and from our cars and actually capture it and bury it underground in so-called carbon capture and storage, you would have to fill up a volume equivalent to Lake Superior every year with liquid CO2. And it can’t leak for 100 years at 1 percent because if it does, after you’ve done that, it’s emitted at the same rate of what you tried to sequester in the first place. So we’d have to have reservoirs with enough integrity that we know won’t leak at one-tenth of a percent a year for 1000 years. That’s what reliable capture and storage technology would entail.
We could do it, but the only proven technology that doesn’t emit CO2 today is nuclear power. I’m not against or for nuclear power. A nuclear power plant produces a billion watts—miraculous machines. We just shut down two in this state. But the earth’s energy meter reads 15 trillion watts, which means we’d have to build 15,000 nuclear power plants. That’s a new nuclear plant somewhere in the world every single day from now until 2050 if you want to meet half of the world’s demand for energy by nuclear power. This isn’t about building ten new nuclear power plants—it’s one every single day. And if we don’t do that, emissions are going to continue to rise and we’re going to get to that new planet. That’s an inescapable conclusion of the laws of physics and chemistry. I didn’t say it was bad; I didn’t say that we should necessarily do it; I’m just saying what the laws of physics and chemistry say the outcome will be.
There is only one big energy source. The undisputed champion of all energy is the sun. More energy from the sun hits the earth in one hour than all the energy consumed on our entire planet in a year. All the oil, gas, coal, hydro, tidal, and nuclear power combined that we consume in a year is equivalent to one hour of sunshine. Nothing else even comes remotely close.
The sun provides us 120,000 terawatts and we need ten or fifteen. We will always have a mix of energy sources, but if you want to play the big card, the one nature gave us to be exploited, it’s the sun. Every country has enough, by far, provided that we can economically exploit it and compensate for a few little technical problems. I’m not the first to say: “We’re all like tenant farmers chopping down the fence around our house for fuel when we should be using nature’s inexhaustible sources—the sun, wind, and tide.”
Why can’t we do it? Well, we’ve just begun to finding out about this thing called electricity. This theme of combustion to get power makes me sick to think about because it’s so wasteful. “I’d put my money on the sun. What a source of power, I hope we don’t have to wait before oil and coal run out before we tackle that.” Anyone know who said that? Thomas Edison in 1931.
That’s what we work on at Caltech. As Arthur C Clarke said, “All advanced technologies, when they get significantly advanced, are indistinguishable from magic.” That’s what we claim to do—magic. There are two ways to store solar energy and both have small problems.
First, nature does it—that’s what photosynthesis is. Photosynthesis gets an A for inspiration (it’s the source of all fossil energy), but a D- for implementation (because it’s not very efficient). In fact, less than 1 percent of the sunlight that hits an acre over a year is actually stored as energy in the fastest growing plants. Then you have to build a farm and convert cellulosic stuff into fuel we can actually use, and put energy in to do that, and it’s all downhill from there.
Solar panels, on the other hand, can be 25 to 30 percent efficient. But they are far too costly, and you would have to put up a million solar roofs every single day from now until 2050 to make enough energy. There’s one other big problem. Gerald Ford, a very wise president on energy said: “Solar energy isn’t something that is just going to come in over night.” He’s the president, he should know.
On the other hand, if you add up all the batteries made in the world in human history they wouldn’t power the US for more than seven minutes. Batteries, as we’ve heard, are not a very energy-dense means of storage. The most energy-dense way is chemical fuels, so why don’t we make chemical fuel directly from sunlight, taking the biggest energy source we have and storing it in the most dense form known to mankind: chemical bonds?
That’s exactly what we’re doing. We’re working on a way to make real photosynthesis where we reduce carbon dioxide to make methanol or gasoline directly from sunlight with no algae, no photovoltaic panels on the roof, no wires, no bacteria. What are the problems? It works; we can make very efficient systems that last twenty years. And we can make systems that are cheap and affordable. But we can only do two out of three at the same time. If you want it to last and be efficient, that’s great, but it isn’t cheap. If you want it to last and be cheap—it’s not very efficient. If you want it to be efficient and cheap, it doesn’t last. Two out of three is good in baseball, but not in technology.
So our vision is basically to make a piece of plastic, that you would roll up like a high-performance fabric, with photoactive materials, just like the chlorophylls that are in green plants, but much better—they are nanofibers of the same materials used in solar panels but instead of powering electricity they directly make chemical fuel. We’ll put in water from water vapor just like a sprinkler system where you water your plants, but instead of just water, you take water and carbon dioxide. We were so proud when we got a shout-out from President Obama during the State of the Union address when he said, “At Caltech, they’re taking water, sunlight, and carbon dioxide and making fuel for our cars.” That’s what we’re trying to do.
It would be like no technology we’ve yet seen, because it’s not like solar panels with electricity—it’s like a Slip-and-Slide that you deploy that makes fuel and that solves the intermittency problem because we can put it in cars, furnaces, turbines, and we can use it to deliver energy to wherever we need whenever we need to. Homegrown energy for all countries—remember, an hour of sunlight for a year’s worth of energy—so everyone has plenty.
The Joint Center for Artificial Photosynthesis is a United States Energy Innovation Hub, through the Department of Energy, in fuels from sunlight. There was a national competition when these hubs were announced, and 20 teams from around the United States, including partners from around the world, all submitted proposals. Eventually the team led by Caltech, that I’m the scientific director of, and our partner, Berkeley National Laboratory, North of Fresno, was chosen.
These hubs are meant to address “the best ideas you’ve never heard of,” as my friend Tom Friedman says. We’re pleased about it too, because they are good ideas. We bring together chemists, physical scientists, physicists, and engineers, and instead of dispersing them into separate units, they all work as a team on this one problem. The scope and magnitude are large enough that we’re willing to roll the dice. This isn’t a $1-million project or a $100,000-a-year grant—our energy innovation hub is funded by a congressional appropriation of $25 million a year for a five-year time period, and assuming that we make progress, which we are, we’ll get yet another five-year renewal.
We’re in our third year, 30 million in, and we have created jobs already in Pasadena and Caltech where we are the nucleus—in your backyard!—of the entire United States’ effort in that technology. We partnered with UC San Diego and UC Irvine because they have skills and expertise in certain areas that we need. But the idea was that this wouldn’t be a dispersed handout of grants but, rather, a focused effort. So while we partner with other institutions, there are only two buildings—one on the Caltech campus and one being built on a Berkeley National Laboratory site. All the work is concentrated only in those two buildings. So all people come together in those buildings, videoconference between those two buildings, and we talk all the time with our partners.
We are doing the basic discovery of the materials needed to get faster, better, cheaper all at once. But at the same time, we’re not waiting for a materials discovery because we’re building prototypes—prototypes we know don’t work today because we don’t have the materials in them to make them work. We were the only team that told people, “We will waste the tax payers’ money, guaranteed.” Because we were the only team that said that we are absolutely going to fail the first prototype because we know it doesn’t work. We’re going to fail the second and third as well, but the only way to get the Wright Brothers’ plane off the ground is to keep trying and learn from the mistakes. That’s what we’re doing. We took the bold approach to not simply take existing technologies and engineer them together in new ways, but to really try to develop a game-changing technology that I think our country is wise to try to do.
Now also, it turns out that now that we are the Joint Center for Artificial Photosynthesis, JCAP, there’s a KCAP—the Korean Center for Artificial Photosynthesis. I just visited them, and they’re copying everything we’re doing and they’re funded at a larger amount of money and jobs than we are. There’s a Chinese Center for Artificial Photosynthesis, a Brazilian center, an Israeli Center, a European center, and I’m going to run out of acronyms soon—all copycats. And although science is an international thing, we still of course have pride to bet on innovation in America, and that’s the bet that we’re happy Congress made.
We built these prototypes with our rapid, prototyping 3-D printer. We don’t know how this thing is going to look; we don’t know whether it should be flat, tilted toward the sun, or with slats that catch the bubbles in certain places, so we build them all with dummy parts. These parts currently aren’t the fastest or cheapest, nor will they survive in the long term, but they still let us test how our design works. This is like building an airplane as it’s taking off on the runway. It’s an accelerated development approach because we think this is an important problem, and the world can’t wait for us to do it stepwise—five years to develop the pieces, five more years to figure out how to put them together, and five years for a prototype. That’s not what our job is.
We think we’re smart, but we’re not that smart, and we’re willing to admit it. So we go back to Thomas Edison and use a big data approach. We have very smart people working on new catalysts, new laboratories, and new materials one at a time, but it might take a person three months to build that new material and test it. It might also take someone—a post-doc, or technical staff—a year to assemble it into a system the first time. We have to do that, but we also can’t leave stones unturned.
So we adapted an inkjet printer. It’s unique in the world because it’s a large-format inkjet printer, that we can get access to the inks, and we made them all the elements that we then squirt out in any composition we want. We use it to make a different compound in every spot. And we built advanced screening tools to measure the properties of every spot. It’s easy for us to make a million spots on a slide the size of your laptop display—that’s how it’s made anyway. It’s easy to wire that up and measure the electrical signal—that’s exactly what your laptop display does. Instead of one person taking a year to measure one new catalyst, we will make a million new catalysts every day. And we quantify a million new catalysts every day, and we have a big database. That’s a good thing, because we can mine it and consult the world. Anyone can look at the data, and we can offer them a prize if they tell us what might make this better than what we made before. Netflix did this—“if you like that movie then you’ll like this movie”—I have seen a lot of better movies that way.
So we make more compounds every day than we’ve made in human history. I don’t want you to think that all our work is done just south of Fresno and in Southern California. We use the Advanced Light Sources at the Berkeley labs; we use the Stanford Synchrotron Radiation Laboratory, not to get radiation, but in order to get beams of light that go very quickly, that can give us the structures of compounds and tell us how these systems work. We are taking advantage of the infrastructure that we’ve built up in our state in order to do this advanced science and nanotechnology.
Since last year there are now 150 good new jobs and training of students and post-docs from around the world, who have come to California to be a part of this project—people from Germany, China, Brazil, and around the United States. It’s a delight because this is what they want to do with their life—to invent something new that the world really needs.
The message is that the world uses a lot of energy—remember: fifteen trillion watts—and it’s not going down because we want economic growth and have population growth. What have we done with respect to CO2 emissions? Well, we’ve actually done a really good job, in the sense that they were growing at 1.1 percent per year, but then we got serious, paid a lot of attention to it, and tripled it! Now they are growing at 3.1 percent per year.
What’s good energy policy? Energy efficiency is the cleanest fuel. It’s the cleanest, cheapest power plant. We should be saving energy like our lives depend on it because they probably do. But don’t be fooled, no amount of energy efficiency ever turned on someone’s light bulb. There are only three big cards that we have to play and that’s fossil, nuclear, and solar. And if we don’t want to make CO2, we had better clean up fossil energy. Nuclear reactors: if we want to build one a day every day forever. Or find a way to use the biggest energy source we have, the sun, but it better be cheap, and it better compensate for the fact that we cannot store and will not have power after four.
I’m going to leave you with a policy decision that someone has to make. Where do we want our planet to go? I never told you this would be good, bad, or that anybody knows what the future will hold (because in fact nobody does). I do know that if we stay on the track we’re on, the laws of physics and chemistry say that that CO2 is going to be there for 10,000 years at levels no humans have ever seen before. And for 670,000 years it has been completely correlated with changes in our climate more than anybody has ever seen so far.
So is failing an option? Maybe. It just depends. It will cost money. It is not the case that it won’t cost money. How much? Nobody knows. We’re just going to have to do the experiment or not do the experiment. This is what we pay our elected leaders to do: make tough choices. People ask me what they can do. You can save energy; you can vote; you can show them that this issue really matters.
So with that, we are so pleased to be working on the problem, to be part of the Southern California community in trying to help represent both Los Angeles County as well as our country in this effort, and thanks very much for letting me share with you this exciting opportunity going on right now at Caltech.
- Log in to post comments